By ALISON WEAVER
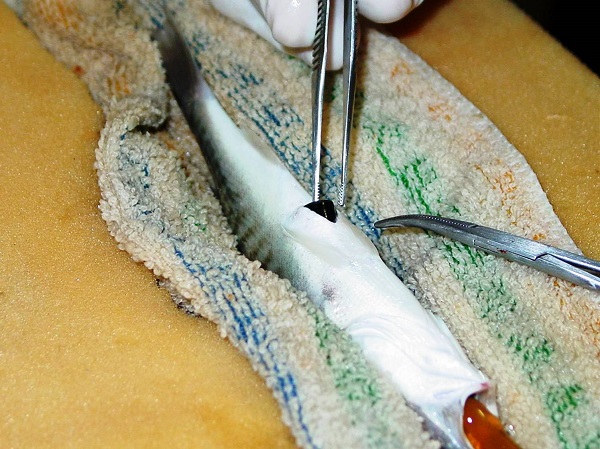
A telemetry tag is implanted in a spot to track its movements in relation to changes in oxygen concentrations in the Neuse River Estuary. Photo by Kevin Craig.
Anyone traveling to the coastal waterfront in New Bern last summer might have noticed some unexpected and smelly visitors. The largest fish kill in nearly a decade left more than four million fish dead and rotting along a 15-mile stretch of the Neuse River estuary between Carolina Pines near New Bern and downstream of Cherry Point.
Fish kills are a very visible sign of stress to the population. In many cases, they are the result of hypoxia, or low oxygen concentrations. Hypoxic water is generally defined as water with less than 2 milligrams of oxygen per liter of water (mg/1).
This ecological phenomenon can be attributed to a mix of environmental and human factors, such as excess nutrients in the water due to increasing urbanization. Scientists and water-quality experts have done extensive work to model changes in water quality in response to this nutrient loading, but the predictions stop there.
“The models don’t tell us how these changes will affect the fish,” says Jim Rice, a biologist at North Carolina State University. Through a series of North Carolina Sea Grant-supported projects, Rice has studied the effects of hypoxia since the late 1990s. Aided by a series of graduate students in the fisheries and wildlife sciences program as well as the biology department, Rice has conducted field and lab studies aimed at quantifying how hypoxia directly and indirectly impacts juvenile fish in the Neuse River.
“It’s important to remember that even in those periods when we aren’t seeing fish kills, there is hypoxia in the river affecting the fish and the habitat in which they live,” he says.
Even when hypoxia is not lethal, it decreases the ability of finfish and shellfish to resist infections, according to Steve Rebach, associate director for North Carolina Sea Grant.
“Our research program has supported projects related to hypoxia because, increasingly, this change in water quality has been responsible for fish kills and ultimately affects the harvest of important species,” Rebach notes.
Rice’s research aims to fill an important information gap by determining how water-quality changes impact the distribution, growth and survival of estuary-dependent fish.
HYPOXIC CONDITIONS
Oxygen dynamics in a river are complex and affected by the physical features of the water body and its biological processes. In the study area, a cross-section of the upper Neuse River estuary approximately three miles wide, the bottom part of the river is filled with saltier water than the fresher, less dense water from the river that floats over top.
The difference in salinities stratifies the water, or creates layers that act as barriers to water mixing. When this occurs, it prevents the water on the river bottom from getting any more oxygen. The isolated bottom water gradually becomes hypoxic as decaying organic matter sucks oxygen from it.
Other impacts to oxygen levels in the river include rainfall and wind. Rainfall can increase hypoxia because it adds fresh water to the system, thereby reinforcing the stratification of the river, along with nutrients that increase production of organic matter. Shallow waters near the shore can provide refuge to fish seeking better oxygen conditions.
Sometimes the wind can create what is known as a seiche (pronounced “saysh”) event. Similar to how water sloshes in a bathtub, the wind pushes oxygenated surface water across the river where it has nowhere to go but down. Hypoxic water near the river bottom is then pushed upward towards the opposite side of the river.
These events can happen quickly, trapping fish and causing fish kills from the hypoxic conditions. “The fish have no time to get out of the way and nowhere nearby to go,” Rice says.
“If the wind is strong enough, it can mix everything up,” Rice says. The system may then become oxygenated and a period of good oxygen concentration ensues.
DEFT SPOT
Rice began a series of experiments in 1998 with various species, including juvenile spot, Leiostomus xanthurus, and Atlantic menhaden, Brevoortia tyrannus. Spot are bottom-dwellers while menhaden are found in surface waters. Both spot and menhaden are estuary-dependent species native to the East and Gulf coasts with commercial and ecological importance, making them particularly good test species.
The experiments were designed to discover how well the fish could detect and avoid hypoxia, and how it would affect their growth and survival. The combined lab and field work yielded surprising results.
“We found that these fish, and even shrimp, are really good at avoiding hypoxic water,” Rice says. They all strongly selected the higher levels of oxygen when given choices in the lab. That preference increased at lowering levels. “They differentiate very well between 1 and 2 mg/1 of oxygen,” he says. “They don’t seem to care quite as much above 2 mg/1.”
Oxygen concentrations had to reach a low level — 1.5 mg/1 — before growth of spot and menhaden was affected, and even lower — below 1 mg/1 — to cause mortality. Other factors, such as warmer water temperatures, can increase the speed at which this occurs. Warm water holds less oxygen and increases the metabolic rate of fish. “The fish need more oxygen to pay the bills,” Rice says.
These lab studies were mirrored by work in the he’d that used hydroacoustic telemetry to track the movements of 21 tagged spot in the Neuse River estuary in relation to changes in oxygen concentration.
“When oxygen was good, they would mosey around in a small area,” Rice explains, “but when the oxygen dropped, they would take off and go farther distances until they finally settled down in another area.” As expected, the spot often settled in near-shore refuges where oxygen concentrations were higher.
Another interesting find was the variability in oxygen concentrations. “We expected things might be relatively stable for a few days, and then a weather front would come through and mix things up,” Rice says. Instead, monitoring in the river found that these concentrations changed greatly in a matter of hours.
CROWDED HABITATS
Even though the spot had proven to be quite good at deftly avoiding hypoxic water, the potential remained for indirect, negative impacts. The fish had to make constant adjustments and spend time in areas with more dense fish populations to avoid impacted areas.
Earlier studies with spot in ponds and cages in the field showed that growth and survival declined as spot density increased. Possible costs from these crowded conditions might be a reduced supply of food for spot, increased activity measurements and less time to feed.
Rice hypothesized that hypoxia will reduce growth in spot by forcing the fish to cram into near-shore, oxygenated refuges. A two-year study monitored water quality at five sites along the Neuse River transect as a first step to learning whether significant density increases occurred in these areas due to avoidance behavior.
Doctoral student Lindsay Glass bottom-trawled the Neuse River in the same three-mile cross section to determine the densities of juvenile spot in relation to the oxygen conditions. Glass conducted short-duration trawls at all five sites. She covered each area three times over the course of a night during the summers of 2006 and 2007 in varying river conditions.
“Our ability to catch fish in the field is not an exact science, but we were still able to detect significant increases in fish density when the refuges were compressed,” Glass says.
Few, if any, fish were present in water with oxygen-concentration levels below 2 mg/l. Densities in near-shore oxygenated refuges nearly doubled when compressed by severe hypoxia.
“The fish are very good at dodging hypoxia, and in fact, there were a few times when Lindsay’s trawls started in low-oxygen water and ended in high, so we know we crossed that boundary of hypoxia,” Rice notes. When that happened, the density of fish would be extremely high; the hypoxic water acted like a snowplow, pushing the fish ahead of it as the boundary moved, he explains.
The studies occurred during a drought, when hypoxic events tend to be less severe. That may explain why periods of habitat compression were generally quite short and occurred just 15 percent of the time. “The fish weren’t stuck in high densities for several days in a row. It really wasn’t costing them very much,” Rice says.
LETTING THE FISH TALK
The rate of growth for juvenile spot is critical to their survival.
“It matters if they are growing 2 percent or 1 percent a day. Even seemingly small changes in growth rates can affect a fish’s survival,” Glass says.
As fish grow, they outgrow certain predators, increase the distance they can travel to find food and boost their ability to tolerate extreme conditions.
Before Rice’s hypothesis can be tested, a finer tool is needed to assess growth due to the dramatic variability in hypoxic conditions over space and time.
“We need a way for the fish to tell us how well they have been growing,” Rice says.
The most recent phase of the hypoxia studies is using three biological indicators to determine how well the fish are growing under different conditions.
“We can’t capture a fish one day, take a measurement, put it back in the water and be able to find and capture it again to determine its change in weight,” Glass says. “We had to find a way to take instantaneous samples of the fish to estimate how well it is growing.”
Glass is using new technologies to help complete the picture. The ratio of RNA to DNA in muscle tissue has recently been used as an index of growth in small fish, based on the notion that the amount of DNA, the primary carrier of genetic information, is stable under changing environmental situations, while the amount of RNA is directly involved in protein synthesis and by inference, with growth and nutritional condition.
But RNA/DNA ratio is affected by other processes besides growth. It can be influenced by temperature and be notoriously difficult to measure.
A second, more direct approach may be found by measuring blood plasma levels of Insulin-like Growth Factor (IGF-1) in the blood, a hormone that is directly involved in controlling growth, according to Glass, who was awarded a Graduate Assistance in Areas of National Need (GAANN) Fellowship in Biotechnology for this novel use of biotechnology. For these studies, the team collaborated with Russell Borski, a fish-growth physiologist at N.C. State. Borski had previously used the hormone in laboratory aquaculture studies. But this project is the first time IGF-1 has been used in the field.
The third indicator being tested is the hepatosomatic index, or the liver weight compared to the overall body weight of the fish. “These fish store energy reserves in their liver, so if they’ve been eating well or if they’ve been starving, it will be reflected in the index,” Glass says.
Rice explains, “These experiments were designed to tell us what kind of tool we have for determining how the fish are doing — do we have a hammer, knife or a scalpel?”
PROVIDING INFORMATION
In summer 2009, Glass took blood, tissue and liver samples from juvenile spot collected at the Neuse River site and in tidal creeks with pristine and urbanized watersheds near Wilmington and Morehead City. At each location, temperature, salinity and oxygen concentration were recorded every 15 minutes using data loggers, and sediment samples were taken to measure the amount of available food.
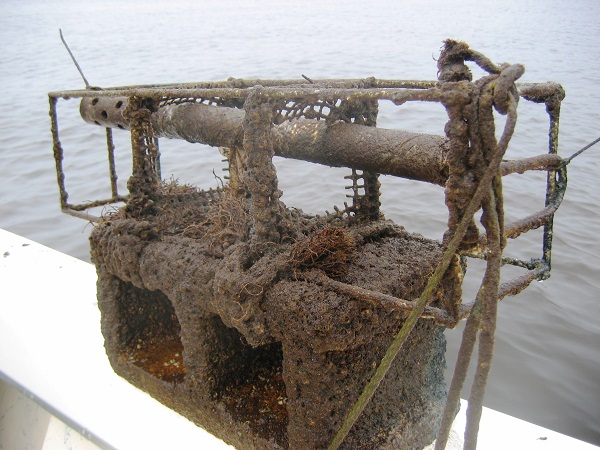
Scientists used a data logger to collect temperature, salinity and oxygen concentration at study sites. Photo by Lindsay Glass.
Meanwhile, back in the lab, Glass took similar samples from spot fed a range of food quantities — from nothing to a limitless supply — to produce a range of growth rates. “Fish in the lab are in an ideal condition — food rains down on them, and they don’t have to worry about predators,” Glass says.
Results from the lab tests hold the key to interpreting index values measured from fish in the field.
“Right now I’m analyzing samples from these ‘calibration’ experiments to figure out how to translate index values from fish in the field into estimates of their growth rates,” Glass says.
Once they know that, Rice and Glass will be able to determine whether changes in water-quality conditions are affecting growth of the fish.
“It is as if we are holding a book written in German, and we are waiting to get our German/ English dictionary,” Rice says.
The results will tell the team how sensitive, or useful, each of the three growth indexes will prove. “This analysis will tell us how closely the indicators track changes in the growth rate of the fish,” Rice says.
A faster response in a particular growth indicator will mean a finer-scale tool. Preliminary results for the IGF-1 indicator, for example, reflect changes after approximately two weeks of feeding.
“If we can only look at water-quality conditions lumped together from the last two weeks, we have a hammer rather than a scalpel,” he says. Data for the other indicators are still being analyzed.
Future experiments will help to develop and refine these indicators. The resulting information will empirically quantify how hypoxia affects fish and provide what Rice calls the “so-what factor” that his department tries to provide in its work. “We are trying to make that connection,” he says.
Resource managers could then use this information to weigh the impact of water-quality changes that affect the fish relative to other factors such as habitat loss or overfishing.
“I want to put my energy into research that can make a difference,” Glass says. “My goal is to provide information that will help managers make informed decisions about our water bodies and fisheries.”
This article was published in the Winter 2010 issue of Coastwatch.
For contact information and reprint requests, visit ncseagrant.ncsu.edu/coastwatch/contact/.